Cardiac Tissue Engineering
Cardiac tissue engineering aims to recreate functional heart tissues for applications in regenerative medicine, disease modeling, and drug testing. Given the heart's complex structure, particularly the alignment of muscle fibers and the coordination required for effective contraction, fabricating tissues that mimic this architecture is a significant challenge. One goal is to engineer tissues that can replace or support damaged heart tissue in patients suffering from cardiovascular diseases.
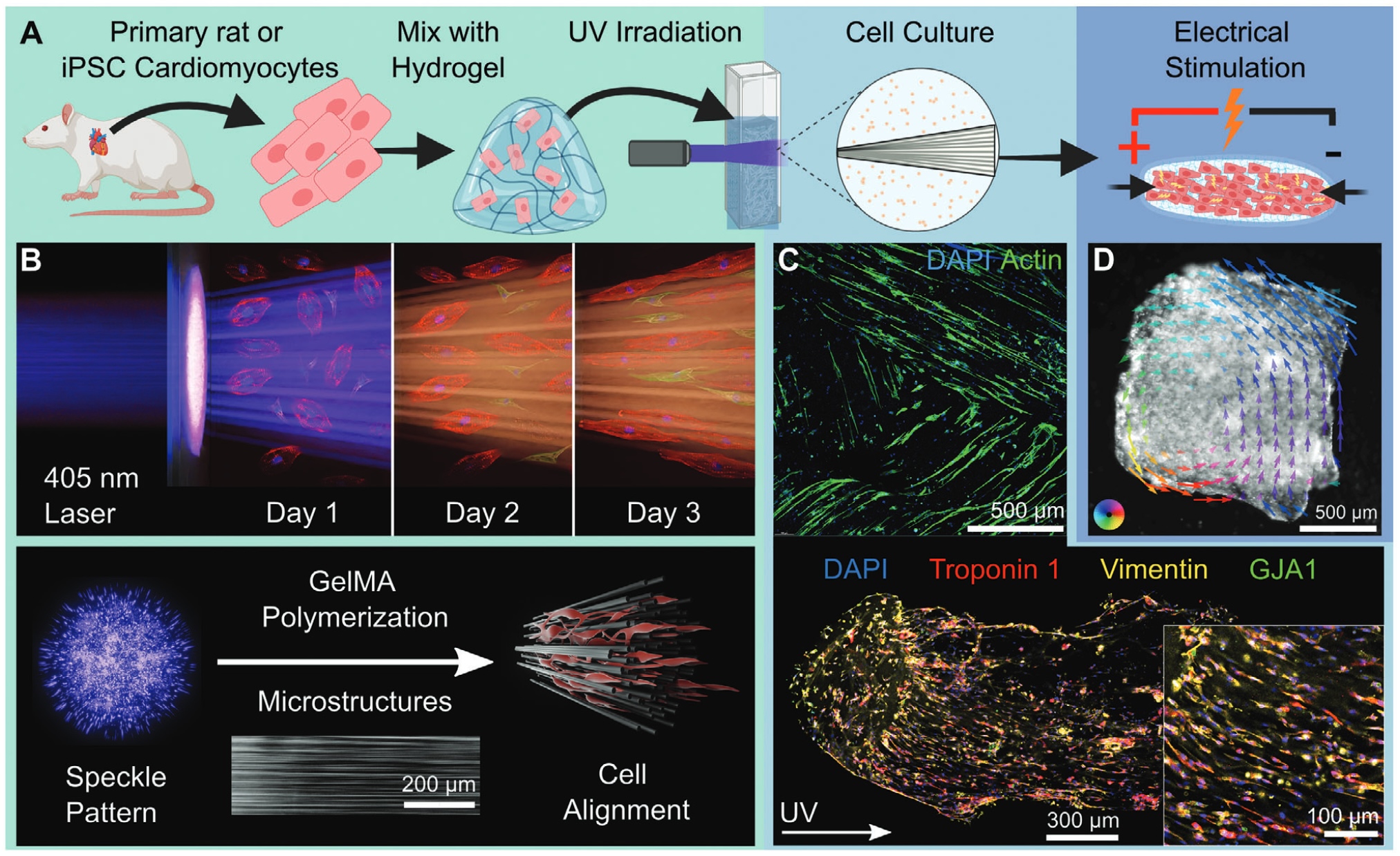
Research Focus: Multidirectional Filamented Light (FLight) Biofabrication
We investigate Multidirectional Filamented Light (FLight) Biofabrication, a novel method that enables the formation of 3D cardiac tissues with multidirectional cellular alignment. This approach uses light to create microscopic structures within hydrogels, which guide cell alignment and enhance tissue contractility. The ability to control the direction and pattern of cell alignment is vital in cardiac tissue engineering, as the heart’s muscle fibers are arranged in specific orientations to achieve efficient contraction.
Current methods for fabricating cardiac tissues often struggle to replicate the high-density cellular organization and alignment required for functional heart tissue. By addressing these challenges, our research aims to provide better models for heart disease studies and therapeutic applications. The ability to create aligned, contractile tissues has the potential to improve cardiac patch designs for repairing heart defects and enhance the development of in vitro systems for drug screening, offering insights into the behavior of cardiac tissues in a controlled environment.
In summary, this research seeks to advance the field of cardiac tissue engineering by developing innovative biofabrication techniques that more closely replicate the structural and functional properties of native heart tissue.
We recently pubished our work in Advance Science: https://doi.org/10.1002/advs.202404509
Research Focus: Using Metamaterials to Reinforce Engineered Cardiac Tissues
Implanting engineered cardiac tissues remains a major challenge, as current patch materials often lack the contractility needed for functional recovery, tend to calcify, and struggle to integrate with the native myocardium. These limitations reduce the effectiveness of traditional patches in restoring heart function.
Our approach involves developing a bioengineered cardiac patch with human stem cell-derived cardiomyocytes embedded in a hydrogel matrix, reinforced with a metamaterial lattice. This design enables precise tuning of mechanical properties, such as stiffness and anisotropy, to closely mimic native cardiac tissue, while providing stability for implantation in the heart.
Our results demonstrate advancements in biofabrication and translational cardiac tissue engineering. By bridging lab innovation with clinical needs, this translational research aims to create a durable, contractile cardiac patch that can enhance the integration and performance of implanted tissues for cardiac repair applications.
We are currently researching this topic in our lab, and will soon communicate our results.